The word “pharmacogenomics” comes from the words “pharmacology” (the study of the uses and effects of medications) and “genomics” (the study of genes and their functions). (1)
Pharmacogenetics (predecessor to pharmacogenomics) dates back more than 2,000 years to observations by Pythagoras, however it was not until the 1950s when some enzyme polymorphisms (e.g., N-acetyltransferase, G6PD) were discovered that the term was coined by Vogel. Pharmacogenetics then went into decline as being too esoteric a subject. In the 1970s the discovery of the CYP2D6 polymorphism and its resultant effect on drug toxicity and response led to many observations of pharmacogenetic-based variations in pharmacokinetics. These and other discoveries and the subsequent ability to genotype led to the term pharmacogenomics.(2)
Pharmacogenomics is the use of genomic and other “omic” information to individualize drug selection and drug use to avoid adverse drug reactions and to maximize drug efficacy. (1)
This essay aims to provide an overview on the principles of pharmacogenomics, how it lead to the evolution of personalised medicine and further directions.
Pharmacogenomics has shown to reduce the risk of adverse events and improve patient health-care outcomes through targeted therapies and dosing. (3)
Every individual has a different genetic makeup, which influences the risk of developing diseases as well as responses to drugs and environmental factors. Genomic differences between individuals are present approximately every 300–1000 nucleotides with over 14 million single nucleotide polymorphisms (SNPs) distributed throughout the entire human genome. Therefore, identification of DNA variants that most significantly contribute to the population variations in each trait is one of the fundamental objectives of genetics.
The understanding of variations in inter-individual drug response behaviours has been greatly improved owing to the rapid developments in pharmacogenomics over the last few years. Each individual in a large patient population responds differently, which possibly explains why a treatment that has been proven efficacious in some patients often fails to elicit adequate responses in others. (4)
Moreover, such treatment failure in the affected patients may cause some serious side effects or even lead to death, which is inductive of individual variability in drug safety and efficacy. The causative factors for variations in drug response are complex and multi-fold with direct or indirect consequences. Among them, stably-inherited genetic factors are the major variables, whereas others include environmental factors like chemicals and radiation exposure, lifestyle factors like drinking, smoking and exercise, and physiological factors like age, sex, liver and kidney function, pregnancy, and starvation. It is evident from previous studies that population variability in drug response is often larger than intra-patient variability (within the same individual at different time points). Drug response of individual patients is primarily determined by the pharmacokinetic and pharmacodynamic properties of prescribed drugs, which is directly or indirectly affected by polymorphisms in drug metabolizing enzymes and transporters. Different populations have varied allele frequencies in genes of both drug metabolizing enzymes and transporters. For precision medicine, the molecular and clinical information is integrated in order to understand the biological basis of disease and develop medications with better outcomes for patients. Therefore, precision medicine will help to improve the selection of disease targets and lead to the identification of patient populations that exhibit better clinical result at normal doses.(4)
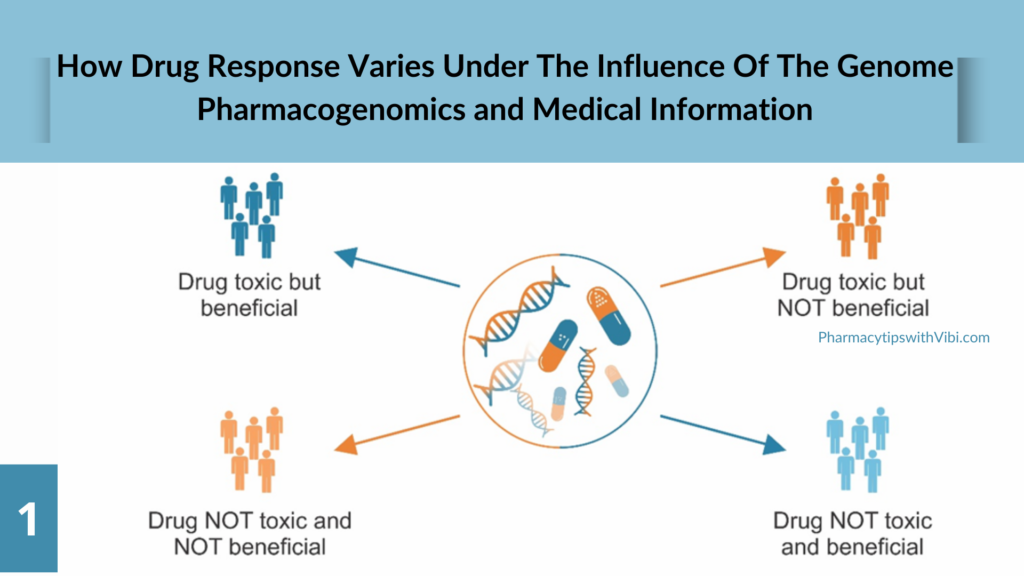
Pharmacogenetic testing helps identify individuals at risk of adverse reactions or non-response to certain medications. A pharmacogenomic test is a genetic test that may look at one gene or several genes for specific changes that impact medication breakdown.
Providers typically use a blood sample or cheek (buccal) swab for pharmacogenomic testing. A provider sends your sample to a laboratory where a technician checks your DNA for specific changes. Which gene or genes they check depends on which test your provider has ordered, which condition(s) they’re trying to treat and which medications they’re considering.(1)
The basic definition of a biomarker is deceptively simple: “A defined characteristic that is measured as an indicator of normal biological processes, pathogenic processes or responses to an exposure or intervention.” (5)
Examples of pharmacogenomic markers include variants in genes like CYP2D6, which affect the metabolism of drugs like codeine, and CYP3A4 which is involved in the metabolism of most drugs.
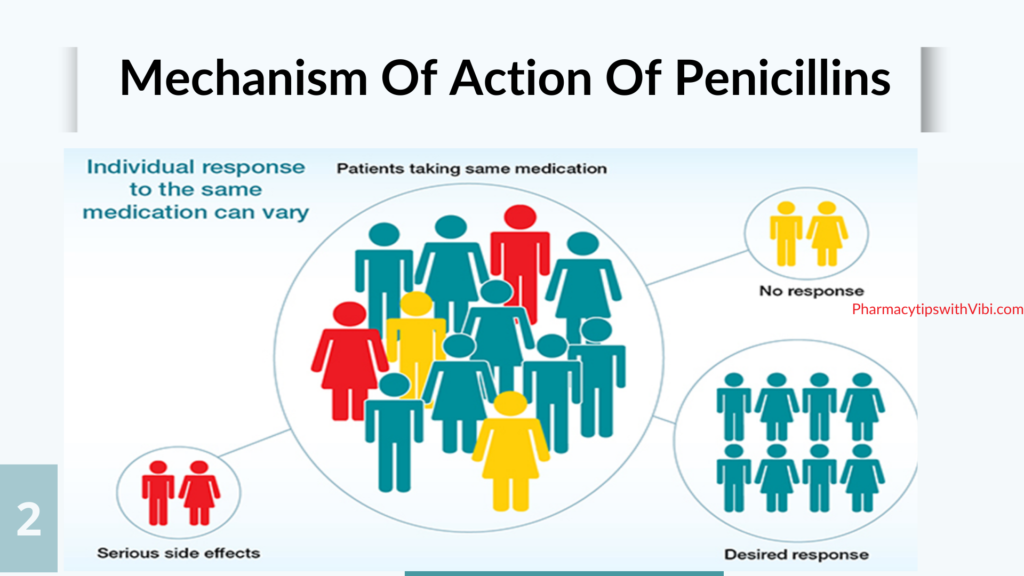
Cytochrome P450 (CYP), which represents a large and diverse group of heme-containing enzyme superfamily, is involved in oxidative metabolism of structurally-diverse molecules like drugs, chemical, and fatty acids. The genetic polymorphism in the genes encoding CYP members was first reported for CYP2D6. More than 100 CYP2D6 genetic variants have been described to date, resulting from point mutations, duplication, insertions or deletions of single or multiple nucleotides, and even whole-gene deletion.
Individuals carrying different CYP2D6 allelic variants have been classified as poor metabolizers (PMs), intermediate metabolizers (IMs), extensive metabolizers (EMs), and ultrarapid metabolizers (UMs) according to the metabolic nature of the drugs and degree of involvement in drug metabolism of these variants. Although constituting only 2%–4% of the total amount of CYPs in the liver, CYP2D6 actively metabolizes approximately 20%–25% of all administered drugs. The drugs metabolized by CYP2D6 include tricyclic antidepressants, serotonin reuptake inhibitors, antiarrhythmics, neuroleptics, and β-blockers.
The percentages of PMs, IMs, EMs, and UMs differ among different ethnicities due to the significant variability in the CYP2D6 allele distribution.(4)
More than 50% of clinically-administered drugs are metabolized by CYP3A4, which is the most abundant CYP enzyme in the liver. Therefore, polymorphisms in CYP3A4 are of great concern in the study of interindividual altered drug metabolisms and related ADRs. More than 26 CYP3A4 variants have been identified and most of these variants are responsible for varied enzyme activities ranging from modest to highly reduced catalytic efficiencies among the affected individuals. Comparatively, high frequencies of allelic variants of the CYP3A4 gene (CYP3A4*2 and CYP3A4*3) were observed in Caucasians whereas high frequencies of allelic variant CYP3A4**18 were observed in Chinese people (Table S1). The clinical consequences of different allelic variants of CYP3A4 are still undefined for many substrates of CYP3A4. Considering the relatively low frequencies, only small changes in the enzyme activity have been caused by CYP3A4*16 and CYP3A4*18 variants. (4)
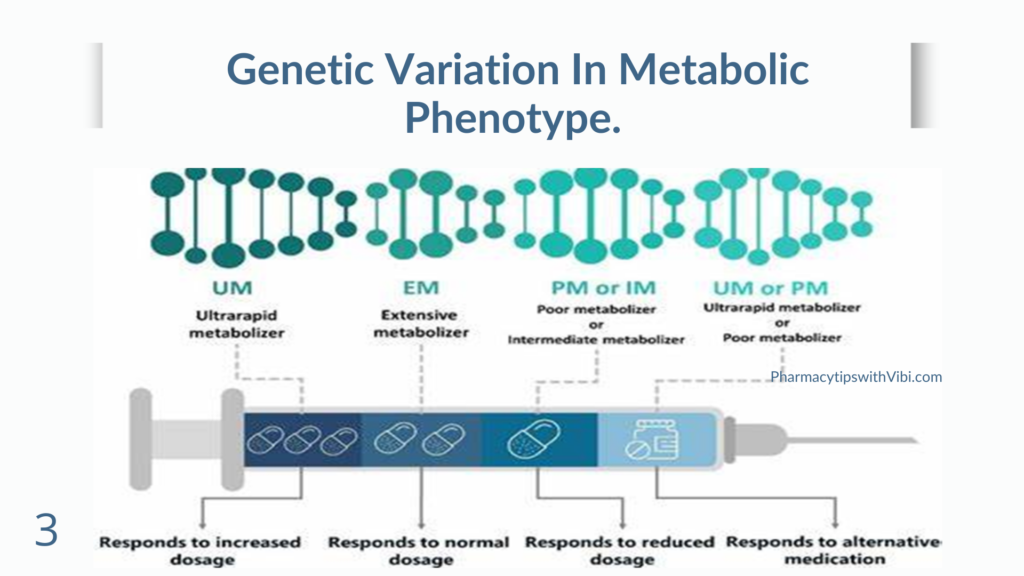
Evolution to personalized medicine is increasingly integrated into clinical practice to guide medication selection and dosing. Pharmacogenomics (PGX ) has been widely studied in cardiovascular medicine. Clopidogrel and warfarin were among the most successful drugs that showed effect of genetic variants on clinical outcomes associated with these drugs.
Clopidogrel is the most widely used P2Y12 inhibitor worldwide especially in the prevention of thrombotic events in patients with acute coronary syndrome (ACS) and/or stroke. Nevertheless, not all patients respond to clopidogrel therapy adequately. This interpatient variability may compromise both efficacy and safety. Clopidogrel is a thienopyridine prodrug that requires hepatic biotransformation to form an active metabolite that selectively and irreversibly inhibits the purinergic P2Y12 receptor and thus platelet aggregation for the platelet’s life span. Fifteen percent of the prodrug gets activated while the remaining 85% are deactivated by esterases. A wide variety of CYP enzymes contribute to the two-step bioactivation process of the clopidogrel; however, genetic studies showed that CYP2C19 mutations have the most pronounced effect on clopidogrel interindividual variability. The wild-type CYP2C19*1 allele yields functional CYP2C19 enzyme with normal metabolism.
One may argue that studying clopidogrel PGX is not that important, since we can use the more potent P2Y12 blockers (prasugrel and ticagrelor) that are also less likely to be associated with interpatient variability. However, studies have shown that clopidogrel is still the most commonly prescribed antiplatelet. This may be due to its reasonable price which may enhance patient adherence. Additionally, clopidogrel is associated with lower bleeding risk compared to prasugrel and ticagrelor.
In addition to clinical studies, several studies assessed the cost-effectiveness of applying genotype-guided antiplatelet therapy compared to the universal use of antiplatelet therapy in patients with MI. Majority of these pharmacoeconomic evaluations have shown that implementing genotype-guided antiplatelet therapy, followed by a targeted administration of the expensive ticagrelor or prasugrel in CYP2C19 no function allele carriers and clopidogrel in noncarriers is the most cost-effective approach compared to the universal use of antiplatelets.
Warfarin For more than half a century, warfarin has been the cornerstone oral anticoagulant medication used in the treatment and prevention of various thromboembolic conditions. Warfarin is a vitamin K antagonist that inhibits the vitamin K cycle by binding to the oxidized vitamin K epoxide reductase (VKOR) and prevents the reduction of vitamin K. Reduced vitamin K is essential for the activation of the coagulation factors (II, VII, IX, and X), in addition to proteins C and S. Consequently, coagulation factors with impaired activity are produced in the liver which leads to a state of anticoagulation. Warfarin is administered as a racemic mixture of two enantiomers (R and S) in almost equal proportions. The S isomer is mainly metabolized by the cytochrome P450 family 2 subfamily C polypeptide 9 (CYP2C9) and it is five times more potent than the R isomer but has faster clearance.60 Among the most important drawbacks of warfarin is its narrow therapeutic index which can mediate serious bleeding adverse events that can even lead to hospitalization and death. Another disadvantage is the inter- and intra-patient variability in the dose required to achieve the optimal anticoagulation response. Dose requirements can vary from 0.5 mg to 20 mg per day. Various studies showed that genetic and nongenetic factors contribute to warfarin dose variability. The most important genes affecting warfarin dose among different populations are the CYP2C9 – a gene that codes for CYP2C9 enzyme which metabolizes and eliminates the more potent S enantiomer of warfarin, and VKORC1 – a gene that codes for the VKOR which is the enzyme inhibited by warfarin.CYP4F2, an enzyme that metabolizes vitamin K, has also mutations in the gene coding for its enzyme that may play a role in warfarin dose variability but to a limited extent and not consistent among all populations. (3)
Advances will require health information technology (i.e., health informatics) solutions and effective EHR systems. With the revolution in whole-genome sequencing and tremendous drop in its cost, genetic data are expected to be present at the tip of our fingers
Some strategies to promote further enhancement of pgx implementation in clinical practice should be put in place to frequently evaluate emerging evidence, continuously audit and evaluate the progress and performance of the program, and integrate research for ethical, legal and social issues. In addition, there is a need to educate HCPs (to enhance their use and understanding of the whole practice) and patients, and to push for more tests’ reimbursement as detailed below.
Education: HCPs and patients are on the receiving end of the clinical PGx implementation process. HCPs, be they physicians or pharmacists, are responsible for the return of results to patients. HCPs and patients are both accountable for reporting back on the outcome of the PGx-guided prescription. They need education to enhance their use and understanding of the whole practice.
Healthcare providers: Survey data have consistently shown that HCPs, despite being generally aware of the importance of PGx and having a positive attitude towards PGx’s ability to improve drug therapy and reduce side effects, few have ordered or recommended PGx testing. This lag in PGx clinical implementation is primarily related to the lack of formal education about PGx testing in medical school and postgraduate studies. These recommendations are crucial, knowing that education and training increase physicians’ confidence to request PGx tests or use such test results if already available before prescribing drugs.
Patients: Patients’ awareness and education are essential drivers for the success of PGx implementation. Available studies suggest that patients have a positive attitude toward PGx implementation and believe in its ability to predict the correct dose, medication efficacy, mild or serious side effects, and explain the family history of medication toxicity. The general public can also understand specific genetic terminology, yet people cannot comprehend underlying concepts and how this may affect their health.
To address this gap, PGx education may be provided through technological tools such as interactive webpages, educational videos, and telehealth sessions. Moreover, written reports should be user-friendly. They can include summaries of genetic results in a tabular or graphical format such as infographics (e.g., human icon drawn as running motion representing rapid metabolizer) and icon arrays (e.g., shaded figures reflecting the proportion of affected out of total). Also, simple drawings of pie charts, risk labels, and tri-color-coding systems for risk assessment (red, yellow/orange, and green for high, moderate, and average risk, respectively) are examples of patient-friendly formats. These methods can generate more confidence in patients toward PGx implementation. Finally, adequate counseling should be provided through written reports or in-person contact by well-trained HCPs or genetic counselors if available. (6)
Eventhough pharmacogenomics holds the promise to improve patients’ response to drug and better health outcomes. The implementation of pharmacogenomic testing in the clinic still faces several challenges, including the following:
- The need for validated markers that have received administrative approval
- Availability of approved assays
- Access to approved assays through Good Laboratory Practice (GLP) or regulated laboratories
- Availability of dosing algorithms that take into account pharmacogenomic tests
- An effective delivery system to transfer test results and dosing recommendations to the clinic (and back again for the fine tuning of algorithms based on postmarket surveillance) (7)
Pharmacogenomics holds great promise for improving patient care and outcomes by personalizing treatment approaches. The integration of pharmacogenomics into healthcare practices has the potential to revolutionize the way we approach disease management. Continued research and implementation of personalized medicine are essential for realizing the full benefits of pharmacogenomics in healthcare.
Bibliographic References
- Professional, Cleveland Clinic Medical. “Pharmacogenomics.” Cleveland Clinic, my.clevelandclinic.org/health/articles/pharmacogenomics.
- “Evolution of Pharmacogenomics.” PubMed, 2008, pubmed.ncbi.nlm.nih.gov/19544663.
- Elewa H, Awaisu A. Pharmacogenomics In Pharmacy Practice: Current Perspectives. Integr Pharm Res Pract. 2019 Nov 8;8:97-104. doi: 10.2147/IPRP.S180154. PMID: 31807435; PMCID: PMC6850702. https://doi.org/10.2147/iprp.s180154.
- Ahmed, Shabbir, et al. “Pharmacogenomics of Drug Metabolizing Enzymes and Transporters: Relevance to Precision Medicine.” Genomics, Proteomics & Bioinformatics/Genomics, Proteomics and Bioinformatics, vol. 14, no. 5, Oct. 2016, pp. 298–313. https://doi.org/10.1016/j.gpb.2016.03.008.
- Califf RM. Biomarker definitions and their applications. Exp Biol Med (Maywood). 2018 Feb;243(3):213-221. doi: 10.1177/1535370217750088. PMID: 29405771; PMCID: PMC5813875.
- Kabbani D, Akika R, Wahid A, Daly AK, Cascorbi I, Zgheib NK. Pharmacogenomics in practice: a review and implementation guide. Front Pharmacol. 2023 May 18;14:1189976. doi: 10.3389/fphar.2023.1189976. PMID: 37274118; PMCID: PMC10233068.
- Marsh, Sharon, and Tibor Van Rooij. “Challenges of Incorporating Pharmacogenomics Into Clinical Practice.” PubMed Central (PMC), 1 Oct. 2009, www.ncbi.nlm.nih.gov/pmc/articles/PMC2806804.
- CDC,Genomics and Your Health, 15 May 2024, www.cdc.gov/genomics-and-health/about/pharmacogenetics.html.